Quick Facts
- Humans use the power of the sun in a variety of ways.
- Solar photovoltaics (PV) directly convert sunlight into usable electricity.
- The PV effect was discovered in 1954.
- Solar PV is decreasing in price and increasing in production efficiency.
- Solar PV panels cost 100 times less than 1977 and a third of their 2008 price.
- Solar PV is within reach for many households to buy or lease…it is not a luxury accessible only for the rich.
- Solar PV may offer a higher financial return on investment (ROI) than many stock market investments.
- Solar PV systems can be connected to the utility grid, can be independent of the grid with a battery back-up, or can function as a hybrid of both.
- When considering the ratios of lifecycle energy outputs (i.e., usable energy) to energy inputs (i.e., lost energy) in powering our economy, solar PV (7:1 and rising) is already competitive with most conventional fossil fuels (as low as 5:1 and as high as 15:1, but declining).
Introduction
Energy is the ability to do work. Our sun is the prime mover empowering the work of life on Earth through radiant light, heat, and magnetism. Since our first use of tools, humanity has tapped the sun’s energy through a variety of technologies.
As omnivores, humans eat the tissues of plants and animals, a store of edible energy derived from the sun. We direct and reflect sunlight to provide natural illumination through windows and skylights. The sun-driven transfer of heat through moving air (convection), solid materials (conduction), and electromagnetic waves (radiation) are forces we partner with (or fight against) to heat water and to condition building spaces for optimal thermal comfort.
This fact sheet focuses on residential building integrated solar photovoltaic (PV) systems, a modern, and increasingly popular, form of harvesting sunlight to work for us at home. The technology has come a long way since the discovery of the PV effect in 1954.
What are Solar PV Systems?
Residential solar PV systems directly convert a portion of light (photo) energy from the sun into electricity (voltage) that can be used at home or sold back to your local utility. These systems are assembled from a set of interconnected components operating in one of three general ways: (1) grid-tied; (2) off-grid; and (3) hybrid.
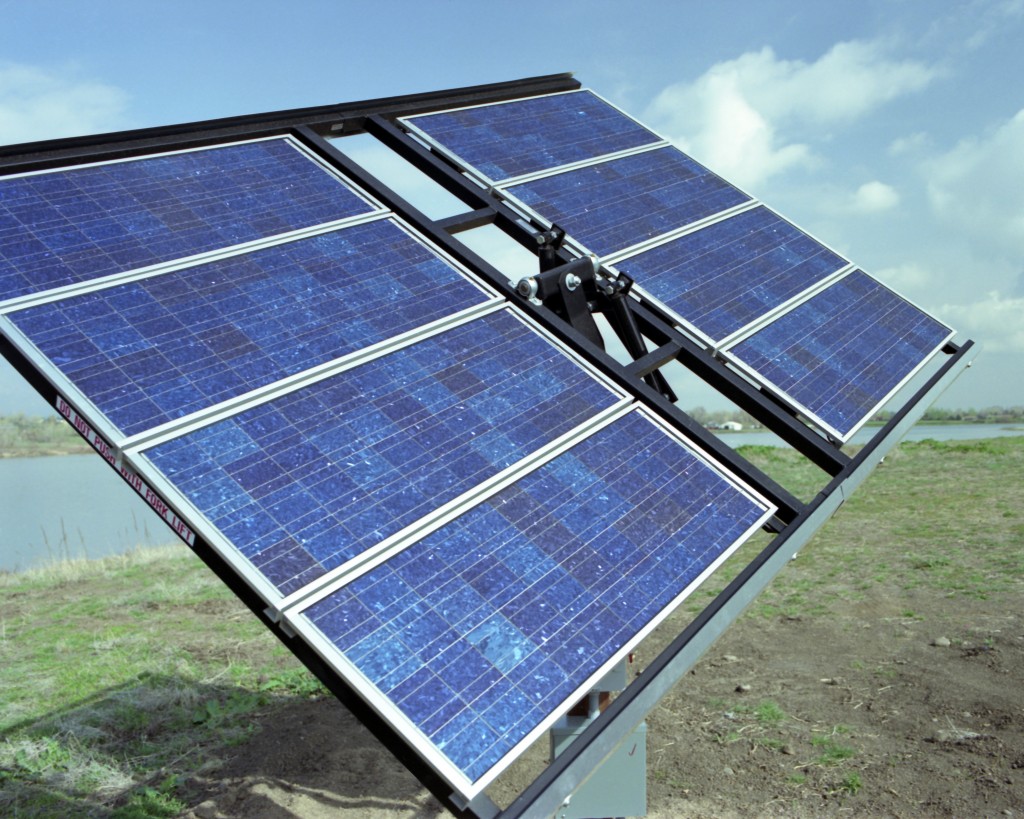
Figure 1. A sun-tracking, free-standing, solar PV array consisting of eight total PV panels, each constructed of 44 individual square polycrystalline PV cells. Credit: Warren Gretz / NREL.
All three solar PV system operational types consist of several major common components. The most recognizable component is the solar PV array (Figure 1), a wired collection of PV panels, each containing one or more weather-sealed PV modules, which are made from smaller PV cells (Figure 2).
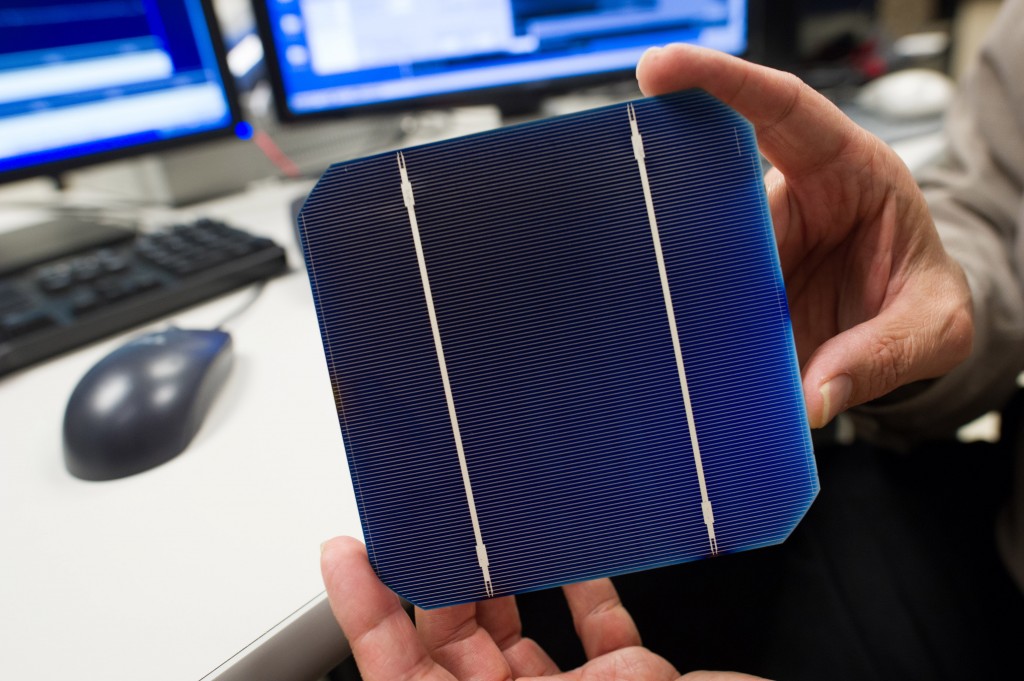
Figure 2. An NREL researcher holds an individual solar PV cell, the smallest light harvesting component in a solar PV system. Credit: Dennis Schroeder / NREL.
An inverter converts the direct current (DC) power from the PV array into the alternating current (AC) power traveling along utility electricity distribution lines and serving our home systems and appliances (Figure 3). Balance of system (BOS) equipment may vary by manufacturer or operational type and typically includes wiring, mounting hardware, DC and AC disconnects, ground-fault circuit interrupters (GFCI), combiner boards, overcurrent protection, and fusing hardware.
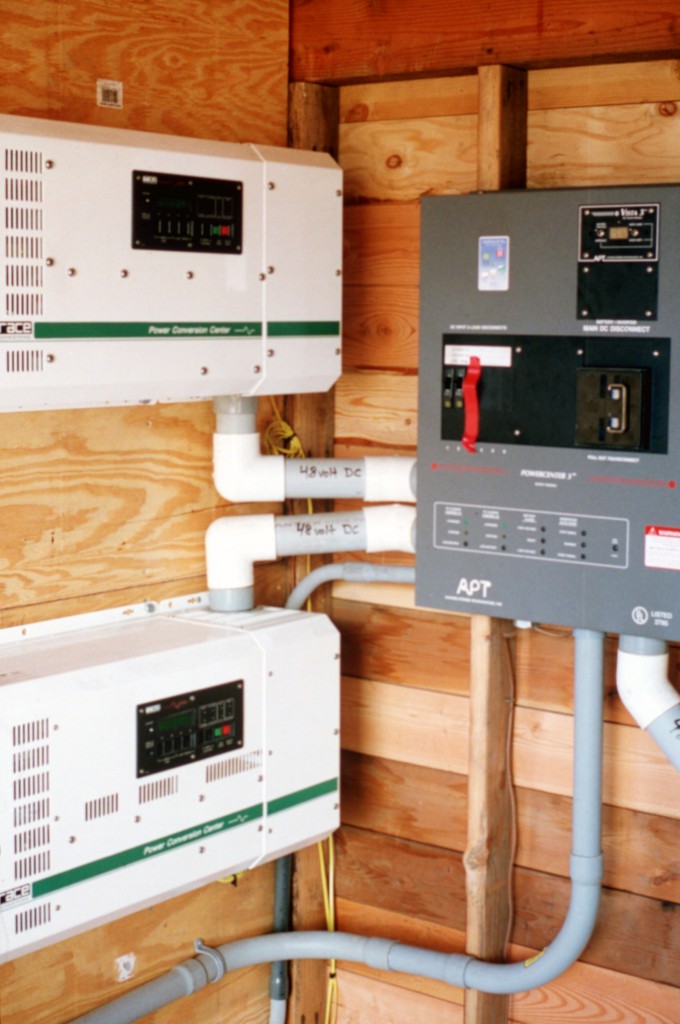
Figure 3. Solar PV inverters and controllers at Channel Islands National Park in San Miguel Island, California. Credit: Kent Bullard / Courtesy of NREL.
Grid-Tied PV Systems
Most residential PV systems are connected to the electric utility grid. Depending on your utility provider, as well as, local, state, and federal requirements, these systems can supply power directly into the home or out onto the grid. In order to protect utility infrastructure and line workers in the event of a power outage, grid-tied PV systems automatically shut down until power is restored to that section of the grid.
Grid-tied PV systems typically offer the fastest rate of return on their investment as many utilities offer incentives. At a minimum, qualified renewable energy generators in Florida owning PV systems up to 2 MW in size may take advantage of the net-metering rules implemented by the Florida Public Service Commission. Additional components common to grid-tied PV systems may include utility switches and utility metering hardware to measure electricity production in kilowatt hours (kWh).
Off-Grid PV Systems
More commonly used in rural areas, off-grid PV systems can supply continuous, stand-alone electricity, day or night, limited only by the size of the integrated battery energy storage. Additional components common to off-grid PV systems may include battery accessories, enclosures, charge controllers, and critical load circuit subpanels.
Hybrid PV Systems
Utility grid interconnection with battery backup is enabled through hybrid PV systems and their controls. These PV systems may require all of the previously mentioned components common to grid-tied and off-grid PV systems. Many off-grid inverters include a hybrid option. Hybrid PV systems typically cost less than off-grid PV systems because the batteries for hybrid systems are sized for emergency need, as opposed to daily use for off-grid systems.
What are the Most Common Solar PV Cell Materials?
Solar PV cells manufacturing is an evolving industry. First generation, flat-plate, crystalline solar PV cells are made from silicon (Si), a semiconducting material common in modern consumer electronic circuits. Though silicon-based PV cells account for nearly 90 percent of PV system sales and silicon is the eighth most common element in the universe and the second most abundant element in the Earth’s crust, it is rarely found in its pure form and must be processed from various silicates. In the early days of the solar PV industry, this refining process and the production of the PV cells was very expensive. But costs have plummeted with manufacturing advancements and as semiconductors have become ubiquitous in so many consumer products. First generation solar PV is typically warrantied for 25 years with lifespans up to 35 years. These solar panels continue to dominate the market because of their combination of low cost, low electricity production degradation rates, and long service lives.
Second generation, flexible thin-film, non-crystalline solar PV cells are made from amorphous silicon (a-Si) or other non-silicon semiconducting materials (e.g., cadmium telluride) just a few micrometers thick (Figure 4). While originally considered a lower efficiency option, thin-film PV cells have been showing significant efficiency improvements both within the lab and within commercial applications during the period from 2011 to 2015 (Figure 5). Additionally, thin-film PV cells offer creative building integration design options (Figure 8 and Figure 9) and bring other benefits such as better tolerances to heat, hail, and shade. However, solar panels using thin-film PV cells have shown evidence of rapid degradation of electricity production and early failure from sun damage to the protective coating and backing. Currently, these are primarily used for specialty situations.
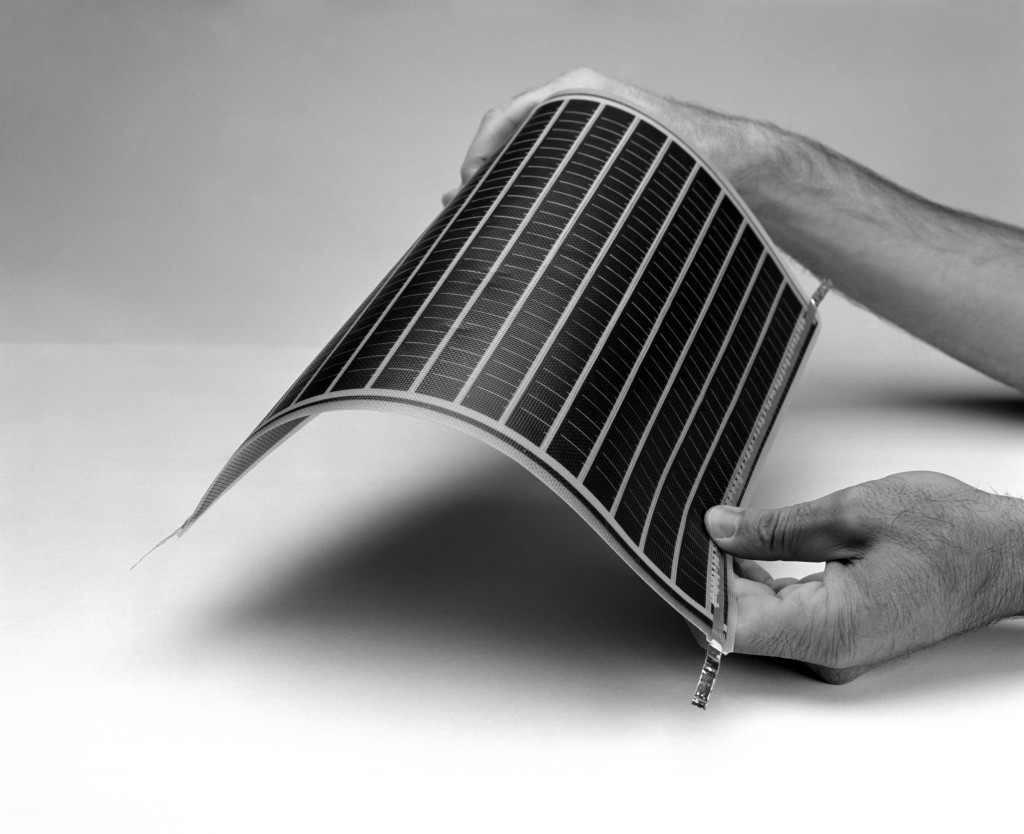
Figure 4. Thin-film solar PV modules, such as this one made with amorphous silicon, can be deposited on a variety of low-cost substrates, including glass and flexible plastic sheets. Credit: Warren Gretz / NREL.
Third generation, non-silicon, solar PV cells are made from emerging materials and manufacturing technologies (e.g., printed solar inks, solar dyes, conductive plastics). In some cases, these novel approaches improve manufacturing productivity. In other cases, they may increase system performance by concentrating sunlight through micro-lenses or mirrors onto smaller sized, but more efficient PV materials. These PV cells are currently being tested for long-term durability and production degradation.
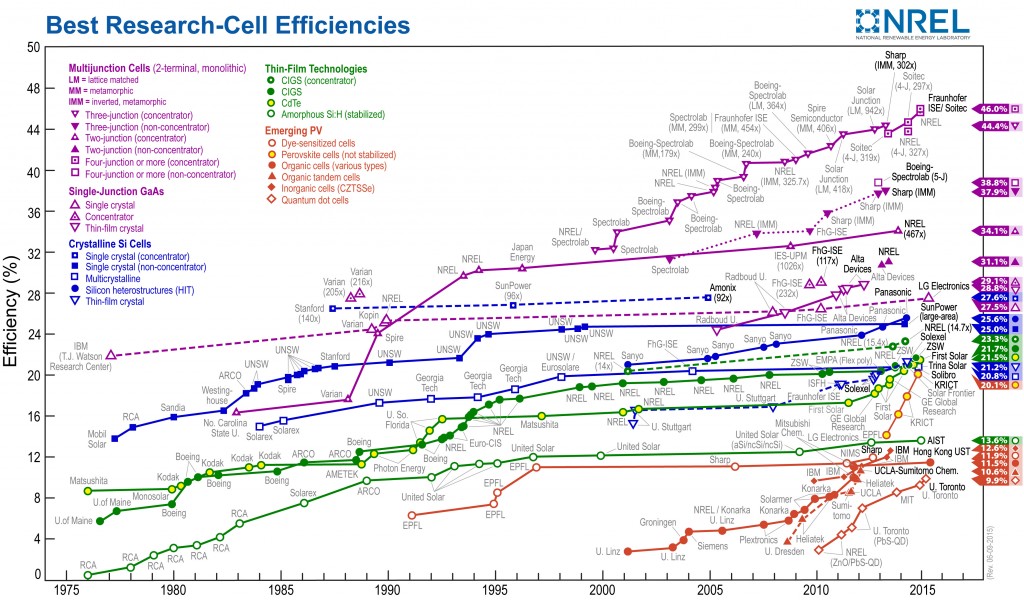
Figure 5. Solar PV cells continue to show efficiency gains within the lab (as evidenced in this chart from Summer 2015), even as solar PV costs for commercial applications continue to decrease in price. Credit: NREL.
Why Consider a Residential Solar PV System?
So long as the sun shines, its light and heat are free sources or renewable clean energy. Solar PV systems produce electricity without the direct greenhouse gas emissions and local particulate air pollution associated with the combustion of fossil fuels such as coal, natural gas, and oil. As our climate changes and our hydrocarbon fuel stocks diminish, renewable electricity becomes an increasingly more important contributing factor to our ecological, social, and economic wellbeing.
The holy grail in the renewable electricity market is grid parity. [1] This is the turning point in the utility marketplace when an “alternative energy” source, most often solar PV or wind power, achieves an unsubsidized, levelized cost equal to the existing electricity retail rate using a fuel mix powered predominately by coal, natural gas, and nuclear energy sources. Another study suggests this point may happen within this decade for both solar PV [2] and wind power, while other studies estimate that we are unlikely to achieve parity until the late 2020s to early 2030s. [3] [4] Beyond grid parity, solar PV systems are attractive for three reasons:
- They provide consumer value;
- They provide a good, and increasing, energy return on investment; and
- They offer net positive life cycle benefits to our society.
Consumer Value
Since wise households live within a financial budget tailored to their income, it may be prudent to ask yourself, “how does a solar PV system investment compare to a few of the most common big household decisions?” These are decisions when an individual, couple, or family must pause for a moment and contemplate commitments that take a longer view than our fast paced daily lives. These financial commitments might include getting married (US $27,021), [5] buying a new vehicle (US $30,303), [6] and buying a new house (US $193,194 in the Gainesville, Florida market in 2013). [7]
Yet, a typical new 5 kilowatt (kW) capacity residential solar PV may cost as little as $17,500 (i.e., $3.50/Watt) installed before subtracting $5,250 for the 30 percent federal tax credit.
A solar PV system this size would produce over $1,100 of electricity per year at an average net metered electric retail rate of $0.17/kWh. Assuming an electricity inflation rate of 4 percent per year, this typical system would provide its homeowner a 25-year average annual return of over 14 percent!
If a homeowner were to roll this cost into a new home purchase or a refinance at a 5 percent interest rate, it would add approximately $65 per month to their 30-year mortgage, yet reduce their power bill by over $90 per month (i.e., every $1 in loan payment is offset by over $1.38 in electricity savings). That’s $25 per month in the homeowner’s pocket. Furthermore, recent studies suggest homes with solar PV systems are realizing premiums of several thousand dollars in resale value versus their non-solar comparables. [8], [9], [10], [11]
Energy Return On Investment (EROI)
While definitions and calculations for energy return on investment (EROI) differ according to system boundaries and other variables, generally EROI is the ratio of units of usable energy outputs for each unit of required energy inputs. A ratio below 1:1 is a threshold at which harvesting and delivering the energy source becomes a burden instead of a benefit to society. An estimate of the domestic oil and natural gas drilled within the United States suggests the oil EROI has declined from greater than 100:1 in the 1930s, to approximately 30:1 in the 1970s, to somewhere between 18:1 and 11:1 by the year 2000. [12]
This means that a barrel of oil in the 1930s was energetically worth nearly 10 times more than a barrel of oil is today. This is because it takes significantly more energy, water, and material resources to harvest the current generation of lower grade oil and natural gas fields than it did in the early days. Furthermore, nuclear electricity EROI is estimated to provide somewhere between 15:1 and 5:1, while the natural gas EROI is around 10:1 and the tar sands and oil shale EROIs are less than 5:1. [13]
On the other hand, the EROI of wind power and solar PV are approximately 18:1 and 7:1 respectively. [14] Regardless of grid parity projections, these alternative energy sources already seem energetically competitive when considering their EROI.
Life Cycle Assessment (LCA)
A life cycle assessment (LCA) is a tool to systematically quantify the ecological, social, and economic impacts of products and services across five major life cycle stages: (1) production; (2) processing; (3) distribution; (4) consumption; and (5) waste conversion. [15] LCAs often take the form of cost/benefit analyses of human goods and services in relationship to one or more of the Earth’s biological, geological, and chemical systems.
An extensive LCA review of solar PV published in 2015 by et al. [16] found polycrystalline PV systems to have an energy pay-back time (EPBT) roughly between 1.0 to 4.1 years. EPBT is effectively the point at which an EROI of 1:1 is achieved. Energy production beyond the EPBT period raises the EROI progressively higher than 1:1 until a solar PV system passes its productive lifespan.
Pacca, et al. [17] (pages 3323–3324) go on to suggest the PV industry is effectively an “energy breeding” system with a net energy ratio (NER or another way of describing the full lifetime EROI) of 8.3 for polycrystalline PV cells and 21.1 for amorphous thin film PV cells. Furthermore, the study [18] suggests the NER “based on a PV system [as the manufacturing energy source] can be 3.7 times higher than the NER based on [manufacturing] electricity supplied by the traditional grid mix, and the CO2 emissions can be reduced by 80 percent.” In other words, the solar PV industry may provide nearly quadruple the NER, with only 20 percent the carbon intensity, of the current electric grid when using solar electricity to manufacture additional solar PV systems. These solar PV advantages are expected to increase for the foreseeable future as innovation continues within both research labs and field installations.
What Factors Limit Adoption of Solar PV Systems at Home?
Despite these strong arguments in support of solar PV systems, there are several common challenges that limit their more widespread adoption for residential applications. While some of these challenges are real, others may be mere misperception.
Initial Cost
Initial system cost is often the primary hurdle. With a 2014 national average cost of approximately $3.50/Watt, homeowners would need about $17,500 in cash and/or financing to install a 5,000 Watt (or 5 kW) sized PV system. [19] For most homeowners, the thought of paying tens of thousands of dollars up front for the next 25 or more years of a portion of your electricity is tough to swallow compared to about $100 for the equivalent amount of electricity consumed as it is used and accrued each month. But given proper financing terms, this hurdle may be smaller than it appears.
Financing Options
Financing options may be hard to find and many homeowners may not meet underwriting standards. With the growth of creative lending models, such as solar PV leasing and property accessed clean energy (PACE) programs, homeowners have more options than in the past. However, finding the right loan product can be an intimidating process. Where no solar PV dedicated loan products exist, homeowners may be constrained by their personal savings and any equity they may have in their home loan-to-value (LTV) ratio. For some homeowners, solar leasing may be attractive because it reduces or eliminates the upfront cost. However, lease agreements should be carefully read because they entail binding commitments over a long-term agreement.
Geographic vs. Site Specific Generating Capacity
Since solar PV systems must pay for themselves over time, optimizing their placement and performance is critical in achieving a meaningful return on your investment. While the American Southwest is an ideal location for solar PV systems, the Sunshine State provides ample opportunity to generate solar electricity with an average production of approximately 3.5 kWh per day per kW of installed capacity.[20] However local conditions matter a great deal. Nearby buildings, urban infrastructure, and vegetation may block access to suitable solar exposure from portions of single days to entire seasons. If and when these potential obstructions take place may also be out of your control.
Grid Connectivity and Energy Storage
Solar PV systems require adequate sunlight and produce less electricity when the sun is low on the horizon (e.g., early morning and late afternoon), when it’s cloudy, or when the panels are covered by dirt, pollen, snow, or other obstructions. Furthermore, solar PV systems produce no electricity during evening hours. As a result, most homes must be “grid-tied” rather than “off-grid.” An off-grid home is powered exclusively by a mix of on-site energy sources, such as a solar PV system, and on-site energy storage, such as a battery back-up system which adds additional cost and space requirements. A grid-tied home is typically powered directly from the local utility infrastructure with the on-site energy sources serving to offset a portion of household energy demand.
Legal Considerations
Access rights and regulations for solar PV systems vary widely by jurisdiction. In some cases, installation may be prevented due to incompatibility with local ordinances and land development codes or due to pre-existing aesthetic standards in homeowners’ association codes, covenants, and restrictions. However, as the general public becomes more “solar savvy,” many cities and states have adopted legislation to protect solar access rights.
Where are the Best Locations for Residential Solar PV Systems?
Solar PV systems are optimized for cool, clear weather with consistent sunlight falling onto the PV cell surface oriented at a 90 degree, perpendicular angle from the sun rays. Ideally, solar PV arrays would be located away from all shading vegetation and structures and would have automated tracking, changing their directional orientation and angle of declination (or tilt) to move with the sun during its daily and seasonal journey across the sky.
However, the cost and complexity of tracking equipment leads most consumers to install fixed, south-facing solar PV arrays. This leads to actual power production somewhat below a solar PV system’s rated capacity. Further reductions in power production come when solar PV is mounted on fixed east-facing or west-facing roof slopes, as compared to fixed south-facing installations.
Beyond the orientation, the angle of declination, and the potential for daily or seasonal shading, there are three common locations for installation of residential solar PV arrays: (1) rooftop mount; (2) free-standing; and (3) building-integrated.
Rooftop Mount
Most residential roofing materials and common roofline slopes allow for rooftop mounting of solar PV arrays using lightweight, windstorm rated, frames and fasteners. Some materials such as asphalt shingles (Figure 6) and metal (Figure 7) are especially well suited to rooftop mounting. Other materials such as clay tile and slate may pose challenges during installation and may increase the risk of roof leaks. With multi-decade lifespans of solar PV systems, it is important to consider the age of your roof before installing a rooftop mounted PV array. Roofs within the last 5 years of their service life may need to be replaced prior to adding solar PV to your home.
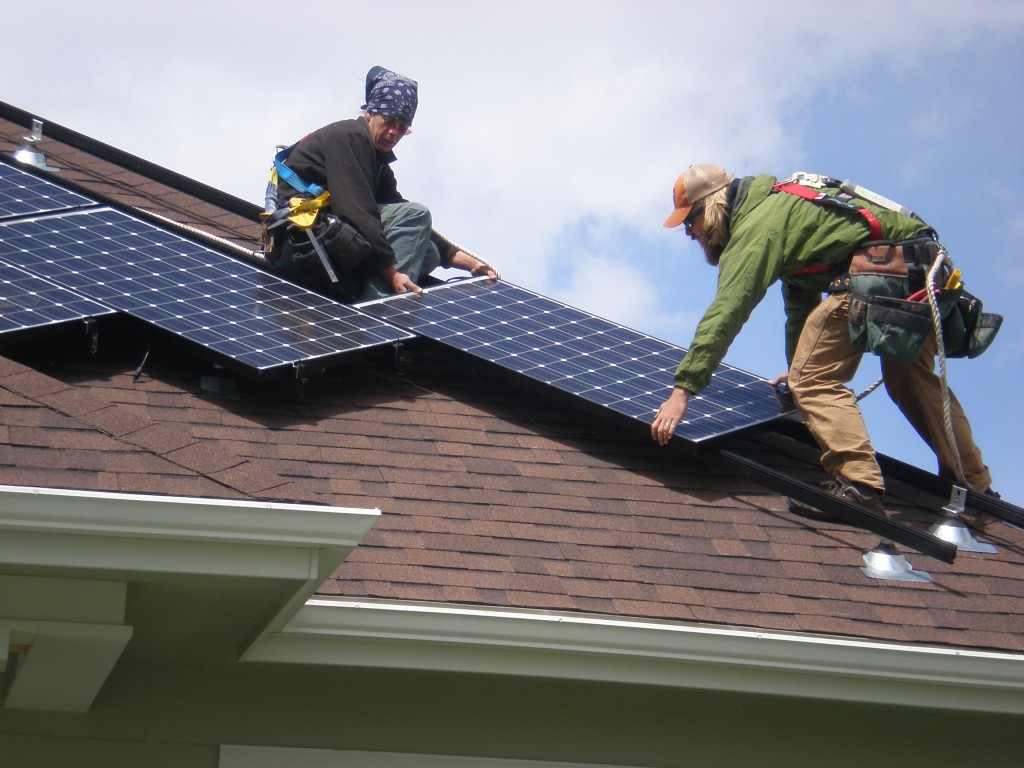
Figure 6. Rooftop mounted PV systems can be attached onto most residential roofing materials, like this asphalt shingle roof, with the proper mounting racks, fasteners, and associated accessories. Credit: Susan Bilo, Outside Photographer / Courtesy of NREL.
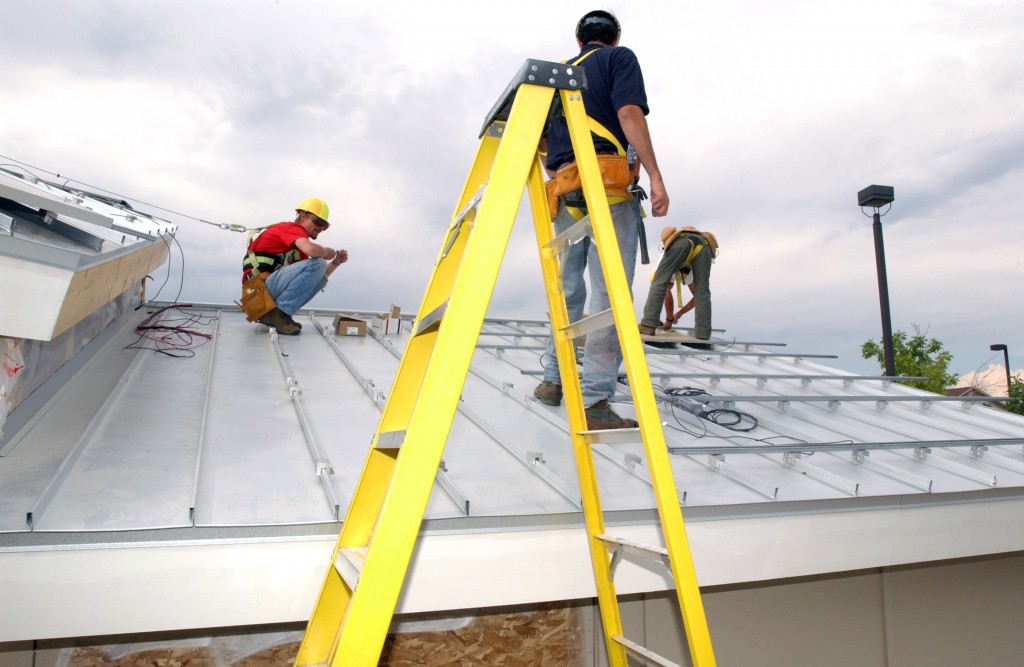
Figure 7. Metal can be a durable, long-weathering, material for Florida roof systems, with an added benefit of reduced leak risks from solar PV installations when using a standing seam as the racks and fasteners “pinch” the seams, rather than “penetrate” the waterproof surface. Credit: Warren Gretz / NREL.
Free-Standing
In some cases the roof of your home may have insufficient solar exposure or may have poor access for installation. Free-standing solar PV systems may be pole-mounted or frame-mounted to the ground at various heights, independent of a building structure (Figure 1). While the mounting poles and frames may increase installation costs, this location type brings benefits such as increased potential to optimize the directional orientation, angle of declination, and solar tracking. Creative homeowners may even integrate solar PV into a free-standing open-air porch or other personal shade structure.
Building-Integrated
Integration of solar PV systems into buildings through the replacement of conventional materials is an interesting option. The most common building integration methods involve solar shingles (Figure 8) and solar windows (Figure 9). However, adoption has been slowed by higher costs, lower production, and untested longevity.
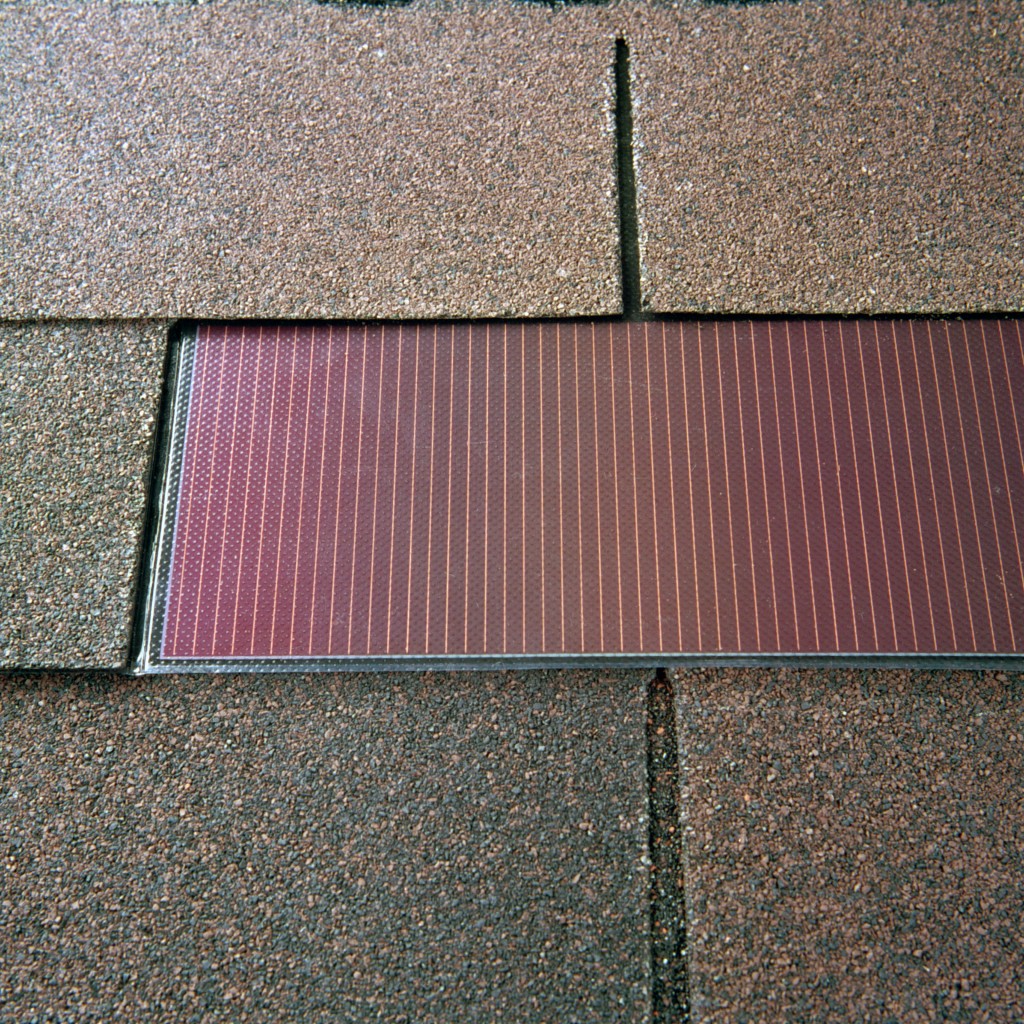
Figure 8. Light, flexible PV roofing shingles for direct building-integrated mounting can blend so well into conventional asphalt shingle roofing that they can be used on new homes where covenants might prohibit more typical rooftop mount solar PV arrays. Credit: United Solar Systems Corporation / Courtesy of NREL.
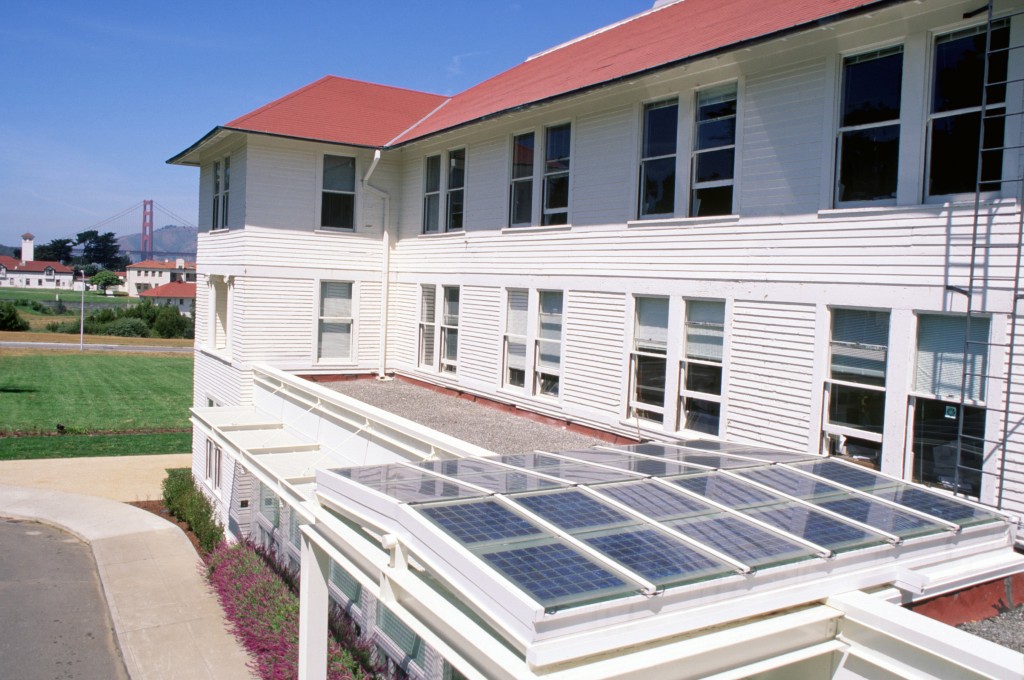
Figure 9. Building-integrated solar PV can also take the form of PV cells laminated directly to windows or skylight glass as shown in the Thoreau Center for Sustainable Development in San Francisco, California. Credit: Atlantis Energy, Inc. / Courtesy of NREL.
Other Location Considerations
While the solar PV array needs to maximize its solar exposure, other PV system components, such as inverters, are typically located in less exposed areas, protected from the sun and rain. Property specific variables, such as, local codes and regulations (e.g., zoning, permitting, utility grid connection, fire, homeowners association), natural hazards (e.g., windstorms, hail), and the risk of vandalism may affect your choice of location and your ability to secure sufficient insurance coverage.
Who Offers Financial Support?
While there are a growing number of solar PV specific financing products (e.g., property assessed clean energy), almost any consumer loan product that can fund home purchases or home improvements can also fund a solar PV system. Possibly, the ideal way to finance a solar PV system is within the primary mortgage of a home purchase. As detailed in the previous example, a solar PV system may be cash-flow positive from day one when tucked within a 30-year mortgage.
For existing homeowners with enough equity in their home, the best rates may be available through refinancing or taking out a property-secured second mortgage (e.g., home equity loans or lines of credit). For homeowners that may be underwater on their primary mortgage, but with stable incomes, good credit, and space within their debt-to-income ratio, an unsecured loan product may be a good option. However, unsecured loans typically have shorter repayment times and higher interest rates which may degrade the solar PV system return on investment.
Incentives for solar PV have historically been available for limited periods of time and have varied across local, state, and federal scales. As the solar PV market has matured and consumer pricing has progressively decreased, many incentives have expired. The best online tool for finding incentives specific to your location is the Database of State Incentives for Renewables and Efficiency (DSIRE).
As of 2015 (and continuing through December 31, 2016), one of the most beneficial incentives a household can utilize to offset the installed cost of a residential solar PV system is the Residential Renewable Energy Tax Credit offered by the federal government. This incentive reduces your solar PV costs by 30 percent through a direct credit applied over one or more tax years until the full credit is achieved. Other local, state, and federal incentives may apply. Visit DSIRE to learn more!
What are the Basic Steps to Installing Solar PV on a Home?
Now that you’ve learned a bit about residential solar PV, you may want to join this growing movement. But what steps must you take to get solar empowered? A recently published, nationally relevant, and extensively peer-reviewed consumer help guide for residential solar PV lists “seven basic steps to going solar” as excerpted and adapted below:
- Get a home energy checkup.
- Complete necessary, cost-effective, energy-efficient home improvements in a logical order.
- Learn to understand your electric (and water) utility bills, how to read your electric meter, how to find available local incentives (tax credits, rebates, etc.), and what rules may apply to you.
- Explore solar system types, your available solar access, and your home orientation.
- Weigh buying versus leasing considerations and verify your financing options.
- Try the Ultimate Solar Calculator from the Institute for Local Self-Reliance (ILSR)
- Consider your technical assistance options, educate yourself on familiar industry terms, and get proposals from several reputable, established solar system providers and installation contractors.
- Ask contractors to show actual cost vs. income examples from existing clients as a point of comparison for their projected outcomes.
- Analyze costs, projected savings, contracts, and warranties to make the best choice for you and your home.
- Remember that periodic maintenance will be necessary over time (e.g., PV panel cleaning, vegetation trimming, inverter replacement).
Find Your Moment in the Sun!
The residential solar PV market is maturing. While solar PV may still be out of reach for some, system specification, financing, and installation solutions are increasingly more tailored to serve unique household needs. This fact sheet offers a stepping stone to those who wish to learn more as we empower our economy from the light of our sun. We encourage you to visit the extensive hotlinks, tools, and resources shared within this fact sheet. Good luck as you find your moment in the sun!
Helpful Solar PV Tools and Databases
Database of State Incentives for Renewables and Efficiency (DSIRE). NC Clean Energy Technology Center.
FHA PowerSaver Lenders. US Department of Energy (DOE) – Office of Energy Efficiency and Renewable Energy.
Find a Florida Solar Energy Expert. Florida Solar Energy Industries Association (FlaSEIA).
Open Energy Information (OpenEI). US Department of Energy (DOE) – Office of Energy Efficiency and Renewable Energy.
Open PV Project. US Department of Energy (DOE) – National Renewable Energy Laboratory (NREL).
PV Value®. US Department of Energy (DOE) – Sandia National Laboratories.
PVWatts® Calculator. US Department of Energy (DOE) – National Renewable Energy Laboratory (NREL).
Registered and Certified Solar PV Modules and Systems for Florida. University of Central Florida (UCF) – Florida Solar Energy Center (FSEC).
Solar Career Map. US Department of Energy (DOE) – Office of Energy Efficiency and Renewable Energy.
Systems Advisor Model (SAM). US Department of Energy (DOE) – National Renewable Energy Laboratory (NREL).
References and Resources
15 Solar Facts You Should Know. Clean Technica.
American Solar Energy Society (ASES).
Florida Solar Energy Center: Solar Electricity Portal.
A Guide to Community Solar. US Department of Energy (DOE) – Office of Energy Efficiency and Renewable Energy.
A Guide to Photovoltaic (PV) System Design and Installation. California Energy Commission Report (500-01-020).
Photovoltaics (PV) Education – A collection of resources for the photovoltaic educator.
Photovoltaics (PV) at Sandia. US Department of Energy (DOE) – Sandia National Laboratories.
Portal: Renewable Energy. Wikipedia.
Renewable Energy Project Finance – Solar Securitization and Public Capital Finance. US Department of Energy (DOE) – National Renewable Energy Laboratory (NREL).
Solar Buzz. An IHS resource.
Solar Energy Resource Center. US Department of Energy (DOE) – Office of Energy Efficiency and Renewable Energy.
Solar Energy and Solar Power Facts. Solar Love.
Solar Power for Your Home: A Consumer’s Guide. LSU Ag Center, Publication #3366. Louisiana Cooperative Extension Service. Reichel, Claudette H. 2015.
Solar Powering America Home. US Department of Energy (DOE) – Office of Energy Efficiency and Renewable Energy.
Acknowledgements
Author: Hal S. Knowles, IIIa
Reviewers: Barry Jacobsonb (2015), Sean Meync (2015 PENDING), and Elias Stefanakosd (2015)
a Program for Resource Efficient Communities, Florida Cooperative Extension Service, Institute of Food and Agriculutural Sciences, University of Florida, Gainesville, FL
b Solar Impact, Inc., Gainesville, FL
c Department of Electrical and Computer Engineering, Laboratory for Cognition and Control in Complex Systems, University of Florida, Gainesville, FL
d Department of Electrical Engineering, Clean Energy Research Center, University of South Florida, Tampa, FL
First published June 2015.
This is a fact sheet produced for the Florida Energy Systems Consortium (FESC). The goal of the consortium is to become a world leader in energy research, education, technology, and energy systems analysis.
Footnotes
[1] Sinke, W.C. “Grid Parity: Holy Grail or Hype? – Photovoltaic Solar Electricity on Its Way to Competitiveness.” International Sustainable Energy Review, January 5, 2009. Retrieved May 1, 2015.
[2] Bhandari, Ramchandra, and Ingo Stadler. “Grid Parity Analysis of Solar Photovoltaic Systems in Germany Using Experience Curves.” Solar Energy 83, no. 9 (September 2009): 1634–44. doi:10.1016/j.solener.2009.06.001. Retrieved May 1, 2015.
[3] Yang, Chi-Jen. “Reconsidering Solar Grid Parity.” Energy Policy 38, no. 7 (July 2010): 3270–73. doi:10.1016/j.enpol.2010.03.013. Retrieved May 1, 2015.
[4] Lund, P.D. “Boosting New Renewable Technologies towards Grid Parity – Economic and Policy Aspects.” Renewable Energy 36, no. 11 (November 2011): 2776–84. doi:10.1016/j.renene.2011.04.025. Retrieved May 1, 2015.
[5] Reaney, Patricia. “Average Cost of U.S. Wedding Hits $27,021.” Reuters. March 23, 2012. Retrieved May 1, 2015.
[6] “Average Price of a New Car?” Forbes, May 10, 2012. Retrieved May 1, 2015.
[7] “Gainesville, FL Housing Data for Builders and Housing Market Intelligence – Housing Prices, Home Permits, and Economic Trends for Gainesville, Florida.” Building Magazine, January 14, 2013. Retrieved May 1, 2015.
[8] Hoen, Ben, Geoffrey T. Klise, Joshua Graff-Zivin, Mark Thayer, Joachim Seel, and Ryan Wiser. “Exploring California PV Home Premiums.” Environmental Energy Technologies Division. Lawrence Berkeley National Laboratory, December 2013. Retrieved May 1, 2015.
[9] “PV Value® Tool.” Sandia National Laboratories. Retrieved May 1, 2015.
[10] “Solar Electric Systems Positively Impact Home Values: Appraisal Institute – AI News Releases – News | Appraisal Institute.” Appraisal Institute: Professionals Providing Real Estate Solutions, October 31, 2013. Retrieved May 1, 2015.
[11] Desmarais, Lisa K. “The Impact of Photovoltaic Systems on Market Value and Marketability: A Case Study of 30 Single-Family Homes in the North and Northwest Denver Metro Area.” Colorado Energy Office, May 2013. Retrieved May 1, 2015.
[12] Murphy, David J., and Charles A. S. Hall. “Year in review—EROI or Energy Return on (energy) Invested.” Annals of the New York Academy of Sciences 1185, no. 1 (2010): 102–18. doi:10.1111/j.1749-6632.2009.05282.x. Retrieved May 1, 2015.
[13] ibid
[14] ibid
[15] Heijungs, Reinout, Gjalt Huppes, and Jeroen B. Guinée. “Life Cycle Assessment and Sustainability Analysis of Products, Materials and Technologies. Toward a Scientific Framework for Sustainability Life Cycle Analysis.” Polymer Degradation and Stability 95, no. 3 (March 2010): 422–28. doi:10.1016/j.polymdegradstab.2009.11.010. Retrieved May 1, 2015.
[16] Bhandari, Khagendra P., Jennifer M. Collier, Randy J. Ellingson, and Defne S. Apul. “Energy Payback Time (EPBT) and Energy Return on Energy Invested (EROI) of Solar Photovoltaic Systems: A Systematic Review and Meta-Analysis.” Renewable and Sustainable Energy Reviews 47 (July 2015): 133–41. doi:10.1016/j.rser.2015.02.057. Retrieved May 1, 2015.
[17] Pacca, Sergio, Deepak Sivaraman, and Gregory A. Keoleian. “Parameters Affecting the Life Cycle Performance of PV Technologies and Systems.” Energy Policy 35, no. 6 (June 2007): 3316–26. doi:10.1016/j.enpol.2006.10.003. Retrieved May 1, 2015.
[18] ibid
[19] Market data for 2014 provided by Solar Impact, Inc.
[20] Lombardi, Matthew, Danny Parker, Robin Vieira, and Philip Fairey. “Geographic Variation in Potential of Rooftop Residential Photovoltaic Electric Power Production in the United States: Proceedings of the ACEE 2004 Summer Study on Energy Efficiency in Buildings, American Council for an Energy Efficient Economy, Washington, DC.” University of Central Florida: Florida Solar Energy Center, August 2004. Retrieved May 1, 2015.